Introduction to Astronomy | | | | | | movie |
Q1: wd size | Q2: sun corpse | Q3: wd color | Q4: ns size | Q5: Pulsar map |
White Dwarfs:
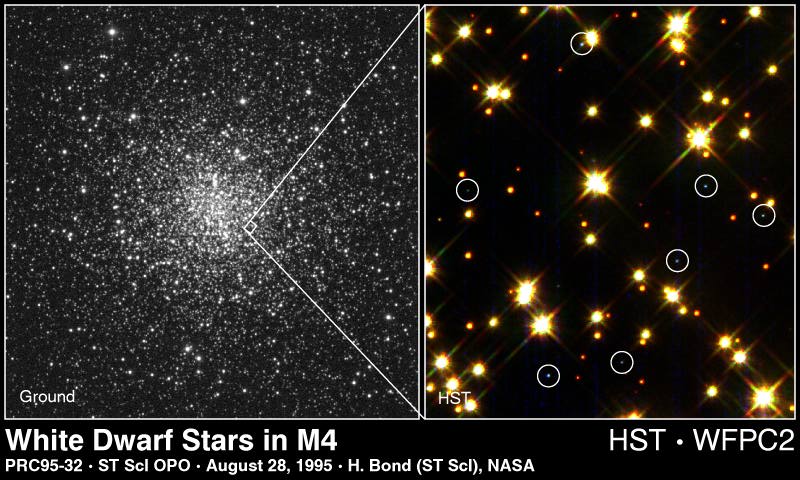
White dwarfs are hot (10,000K), dim stars. This at first seems like a contradiction all by itself, since hot things are usually quite bright. However, white dwarfs are also very small...
They are made mainly of electron-degenerate matter, which means that the pressure does not respond to an increase in temperature in the usual way. This causes white dwarfs to have the odd property that if their mass increases their radius decreases. Stop and think about that for a second. Most things in regular life don't operate that way. If a dog is larger, it has more mass, as a rule. Bigger slugs are more massive than smaller slugs. For example:
- a 0.5 MSun white dwarf has a radius of 1.5 REarth
- a 1.0 MSun white dwarf has a radius of 0.9 REarth
- a 1.3MSun white dwarf has a radius of 0.4 REarth
This is pretty weird. The largest possible white dwarf mass is 1.4 M
Sun. This is called the Chandresekhar limit, and is the most mass that the electron degenerate core can hold up. Objects with larger masses either lose the mass while still on the Asymptotic Giant Branch, or contract still further to become neutron stars or black holes. Once a white dwarf has collapsed to its final size, it has no more nuclear fuels. The interior, which still has heat from before, radiates that heat away slowly over time. This is similar to what happens to a big lump of steel when you take it out of the smelter. It gradually cools down, fading from white hot to blue hot to yellow to red, and eventually becomes a cold, steel-colored lump. The star, just like the steel, remains the same size the whole time. As the star cools, its luminosity decreases, just as you'd expect. This process slows over time, so that the dimmer it gets, the slower it gets dimmer... A 0.6 M
Sun star will:
- drop to a luminosity of 0.1 LSun in 20 million yrs.
- drop to a luminosity of 0.01 LSun in 300 million yrs.
- drop to a luminosity of 0.001 LSun in 1 billion yrs.
- drop to a luminosity of 0.0001 LSun in 6 billion yrs.
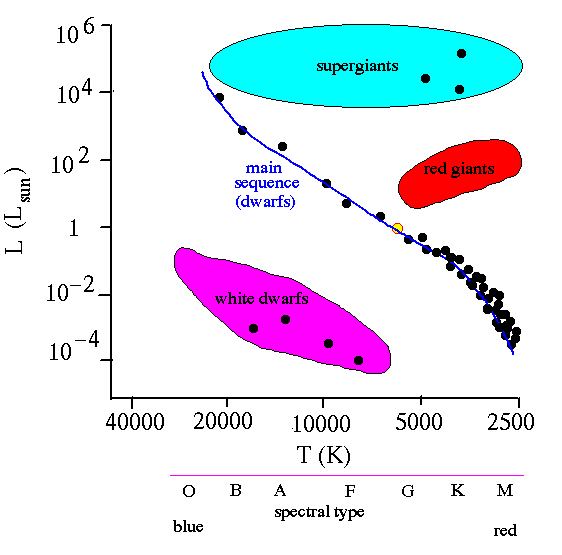
At this point, the temperature (and therefore the color---why?) of the star are about the same as the Sun's, but the luminosity is much less, because it is so small. White dwarfs are found at the bottom of the HR-diagram, with their temperature depending on how much cooling they have done.
Where do these objects come from? They are the central stars of planetary nebulae, and their final mass depends on their initial main sequence mass:
- a 2-8 MSun main sequence star produces a 0.7-1.4 MSun white dwarf.
- a < 2 MSun main sequence star produces a 0.6-0.7 MSun white dwarf.
- a < 1 MSun main sequence star produces a < 0.6 MSun white dwarf.
No white dwarfs with masses less than 0.6 M
Sun yet exist. The Universe is not yet old enough for stars of less than 1 M
Sun to have evolved to the white dwarf stage.
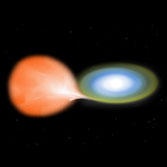
Single white dwarfs can do nothing at this point except continue to cool until they are dark, cold clumps of mass, just sitting there in the galaxy. Some people call this endpoint a 'black dwarf'. They are the logical consequence of a single white dwarf cooling down. (A white dwarf that is part of a binary system, however, can produce a Type I supernovae, which is somewhat more exciting than just cooling down forever and ever. See picture at right.)
Neutron Stars
Neutron stars are produced by main sequence stars of 8-25 M
Sun. These stars become supernovae, lose a lot of their envelope, and leave a degenerate neutron core behind. We are once again talking about degenerate matter, and so the star gets smaller when it is more massive. For a sense of scale: a 1.7 M
Sun neutron star has a radius of ~10 km! That's smaller than Seattle! The maximum mass of a neutron star is probably a bit less than 2.7 M
Sun, but our understanding of the gravitational physics involved in these weird objects is not quite good enough to be certain.
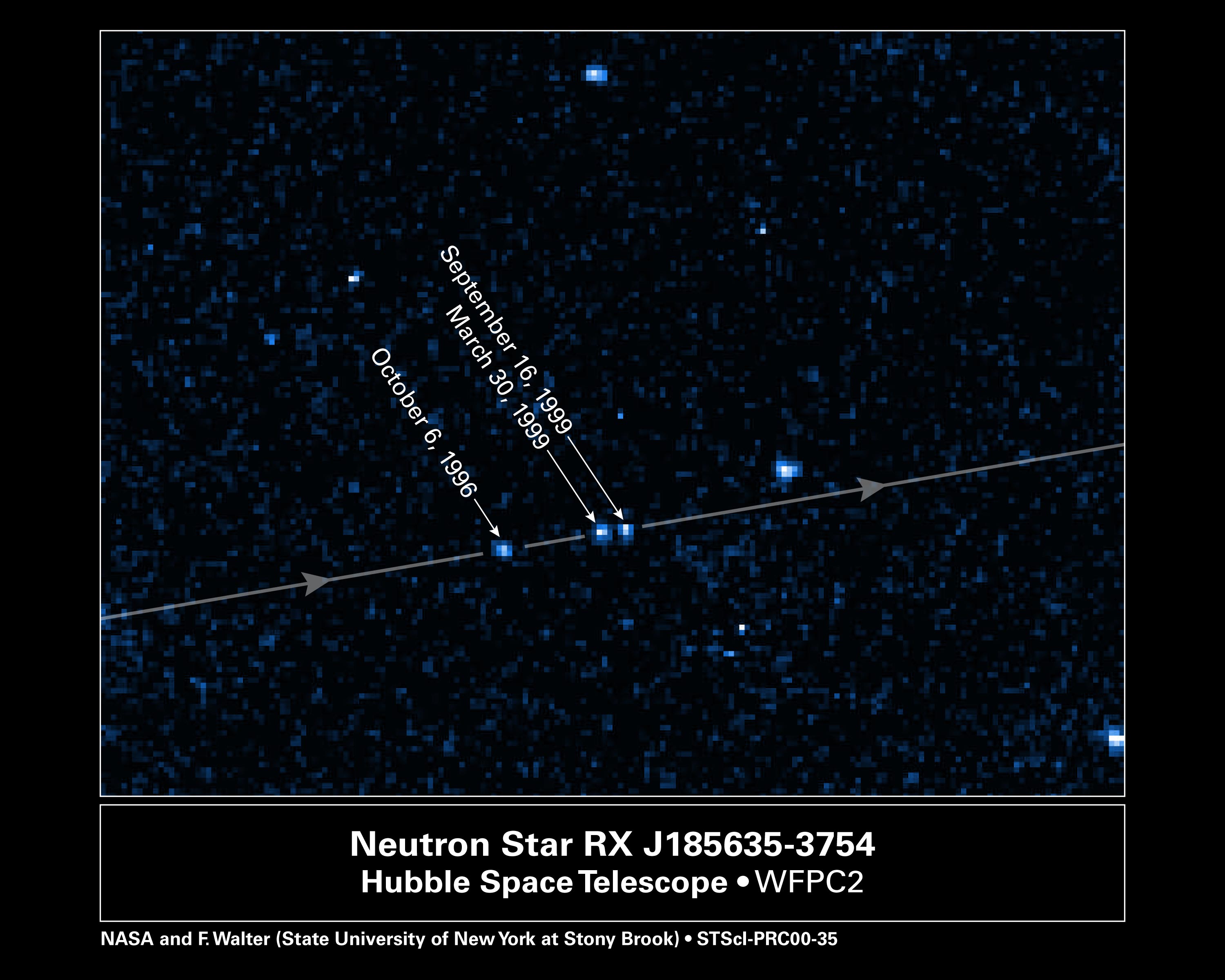
The Motion of RX J185635-3754 - The Nearest Neutron Star to Earth, was captured by Hubble Space Telescope from 1996 to 1999. This neutron star is 200 light years from the Earth. These neutron stars collapsed from larger stars, and during that collapse, angular momentum must have been conserved. That means the stars are rotating
very rapidly, about 1000 times per second! They also have large magnetic fields, due to the collapse and compression of the original magnetic field of the star.
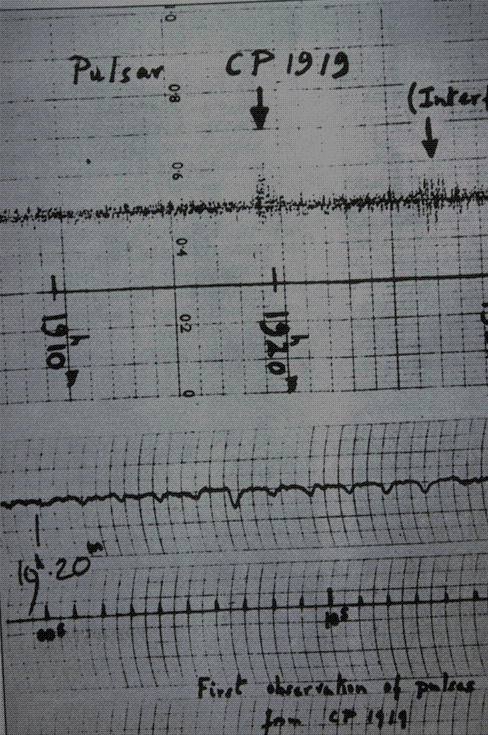
One special type of neutron star is the
pulsar. In 1967, at Cambridge University, a graduate student named Jocelyn Bell was working for this guy named Hewish, setting up 2048 radio antennas all over the English country-side. Once she had this large radio telescope working, she started collecting data, which in those days was recorded on a chart recorder. (Chart recorders spew out reams of paper, kind of like one of those old-fashioned ticker-tape machines. Along the paper is the time axis, and across the paper is the strength of the radio signal.) She noticed, if you can believe this, that every
400 feet (pace this off for yourself sometime...), there was a little fuzzy blip in the signal on her paper. Hewish thought she was mad. But she was persistent, figured out that it came from the sky (how would you do this?), and finally gathered enough data that he started to take it seriously. Their first thought was "little green men", which made Bell pretty mad, because it would get in the way of her finishing her thesis so she could stop working for Hewish. But then, they found another one, and another one, and it seemed pretty unlikely that little green men would be trying to communicate from all these different places in exactly the same way, at exactly the same time. So another guy came up with the idea of a spinning neutron star. (By the way, Hewish got the Nobel Prize for the discovery, and didn't share it with Bell. Or with the guy who figured out it was a neutron star.) What Bell had discovered was a
pulsar.
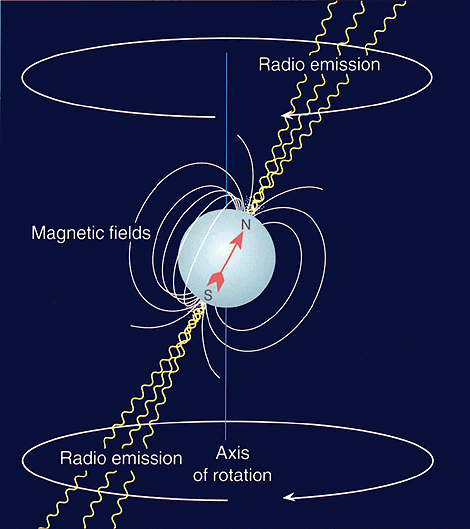
Pulsars are spinning neutron stars, with the magnetic field axis not aligned with the rotation axis (this happens with lots of things, our own Earth is one example). Along the magnetic field lines that come out of the pole are many spiraling charged particles, which emit radiation. When one of these poles is pointed towards us, we see a flash of light. When neither is pointed towards us, we don't see any light. The whole system functions very much like a light house. There can be variations in these pulses. Sometimes individual pulses are shaped differently, and sometimes there are quiet periods with no pulses at all. This implies that the charged particles come out of the star slowly over time. A movie of the variations of the nebula surrounding the pulsar at the center of the crab nebula has been created using both X-ray (left) and optical (right) images. The spinning of the pulsar slows down over time. It takes about 30 million years for the rotation to slow from 0.001 seconds to 2 seconds. This is pretty short compared to the age of the Universe, so you might expect to see lots of these with periods longer than 2 seconds. However, no pulsar has ever been observed with a period longer than 5 seconds. This implies that there must be some sort of shut-off mechanism for the charged particles as the star slows its spinning. It is possible to make a map of our location in the galaxy using pulsars. Cataloging where we see pulsars with particular periods will identify not only a unique location, but also a unique time. This has been done, and was sent on the record that went out with Voyager. If any aliens find it (and can figure out what the heck we wrote), they will know exactly when and where the spacecraft originated!
0 Response to "What Happens if White Dwarfs Continue to Cool Off"
Post a Comment